Our Research
CNRL
Catalysis & Nanotechnology Research Laboratory
Welcome to the Catalysis and Nanotechnology Research Laboratory (CNRL) at Kansas State University. Our research program focuses broadly on rational catalyst design for controlled and scalable growth of carbon nanotubes (CNTs) and related nanostructures via catalytic chemical vapor deposition (CVD). We are particularly interested in understanding nanoscale phenomena and processes, as well as designing game-changing, well-tailored, multifunctional hybrid nanostructures for application in heterogeneous catalysis, energy storage, and environmental remediation.
1. Efficient and Controlled Synthesis of Carbon Nanotubes
1.1 Growth of CNTs Using an Industrial Gaseous Waste as a Feedstock
Although significant progress has been made in the past two decades in scaling up CNT production via catalytic CVD, the process still faces key challenges such as poor yield due to short catalyst lifetime, low catalyst nucleation density, slow production rate, variations in CNT properties, and high cost of CNTs. We are developing a robust process for scalable, efficient, and low-cost production of CNTs of controlled properties. The goal of this effort is to develop the fundamental understanding required to couple CVD to the waste stream of the Fischer-Tropsch synthesis (FTS) process for scalable, continuous, and controlled growth of high-quality vertically aligned CNTs (or CNT carpets) on traditional and nontraditional catalyst substrates (Figure 1). The central hypothesis is that use of the gaseous product mixture of FTS (FTS-GP), usually consisting of C1-C4 hydrocarbons and unconverted CO and H2, as a feedstock during CVD will dramatically enhance CNT growth efficiency by simultaneously providing a high flux of carbon atoms to the catalysts and protecting them from deactivation via oxidative removal of amorphous carbon by water generated on site (see reaction in Figure 1).
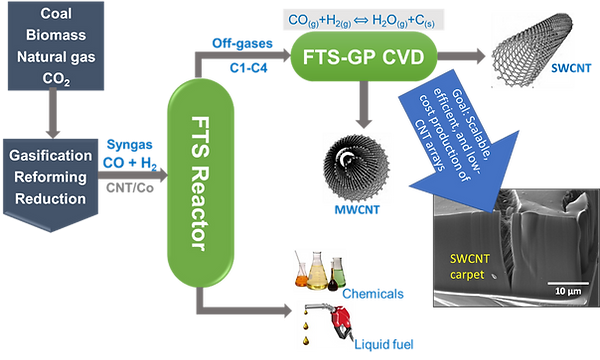
Figure 1. Schematic illustration of the concept of FTS-GP CVD for scalable, efficient, and low-cost production of CNT carpets on nontraditional substrates utilizing a gaseous waste product from FTS; carpets consist of predominantly single-wall CNTs (SWCNTs) or multi-wall CNTs. (MWCNTs).
The unique composition of FTS-GP makes FTS-GP CVD well-adapted for low-temperature growth due to the generation of free radicals from unsaturated hydrocarbons that attack the more-stable saturated hydrocarbons. If successfully coupled to the waste-gas stream of FTS reactors, FTS-GP CVD is expected to promote large-scale production of single-wall CNTs (SWCNTs) or multi-wall CNTs (MWCNTs) that will meet the ever-increasing global demand and minimize the amount of flue gases in oil refineries, thus enhancing environmental protection. Large-scale synthesis of CNTs has the distinct possibility of producing broad implications in multiple applications such as catalysis, clean energy, and thermal management.
1.2 Mechanistic Understanding of CNT Growth
Many important applications of CNTs require their synthesis on nontraditional substrates with exquisite control of their geometry. We are developing an in-depth fundamental understanding of the nanoscale phenomena occurring during the growth process that will guide the design of efficient catalysts. The approach involves a variety of surface analytical techniques, including in situ and ex situ electron microscopy and advanced spectroscopy, to probe the catalyst and CNT growth kinetics. In particular, due to the breadth of parameters that affect CVD growth of SWCNTs, rapid experimentation is necessary for effective optimization and elucidation of the role of key factors in the growth process. We are employing an autonomous research system (ARES) at the Air Force Research Laboratory — an automated, high-throughput, laser-induced CVD system with in situ Raman spectral feedback — to measure SWCNT growth kinetics and chiral selectivity of SWCNTs. The body of knowledge developed from this study is expected to allow for development of growth maps and rational guidelines for design of recipes for controlled growth of SWCNTs.
2. Photocatalytic Air Purification
Photocatalytic oxidation using semiconductors as catalysts has become an appealing approach as a green technology for environmental remediation. Nanocarbon-supported TiO2 composites have attracted significant interest due to the ability of nanocarbon to induce synergistic effects between the carbon phases and TiO2 that inhibits the recombination rate of the photo-generated electron-hole pair and red-shifts the photo response to the visible region. CNTs are of particular interest as photocatalyst supports because they can be either semiconducting, semi-metallic, or metallic, depending on tube helicity and diameter. Importantly, the presence of semi-infinite states in the conduction band of SWCNTs and the possibility of transferring electrons from the nth von Hove singularity to TiO2, as shown by DFT calculations (Long, J. Phys. Chem. Lett. 2013, 4, 1340), are quite intriguing and open up opportunities not yet exploited for tailoring the function of CNT-TiO2 heterojunction. We are interested in understanding the nanocarbon enhancement mechanisms and developing a pathway for the synthesis of low-cost and scalable visible-light-active nanocarbon-based/TiO2 coatings on a variety of common substrates for outdoor oxidation of pollutants as schematically shown in Figure 2.
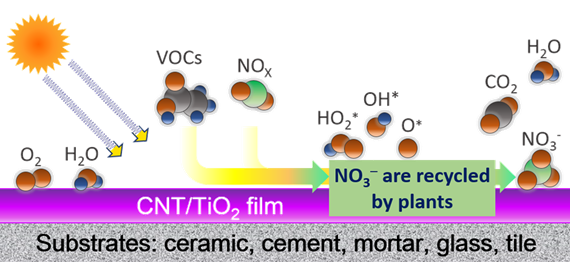
Figure 2. Schematic illustration of CNT/TiO2 photocatalytic coating for outdoor air purification.
3. Carbon Nanotubes as Support for Transition Metal Catalysts
Inspired by the unique morphology, extraordinary material properties, and tunable surface chemistry of CNTs, this study explores their use as catalytic templates for synthesis of new-generation catalysts with high performance (high activity, long lifetime, and high product selectivity) for important reactions such as FTS and dry reforming.
Efficient catalysts are required to convert natural gas to higher hydrocarbons. Such superior catalysts will require new support characterized by high mesoporosity and thermal conductivity, as well as improved chemical stability. Products of FTS usually follow the Anderson-Schulz-Flory (ASF) distribution, which imposes a limitation on the C5+ selectivity even at optimum probability of chain growth; thus yield of low-molecular-weight gaseous products, currently being utilized as a feedstock for CNT growth, is unavoidable. Our goal is to establish a closed-loop material system for FTS as illustrated in Figure 3 whereby the FTS-GP in process (A) is used as a feedstock for mass production of CNT arrays in process (B), and the grown CNTs in turn serve as support for FT catalysts in process (C).
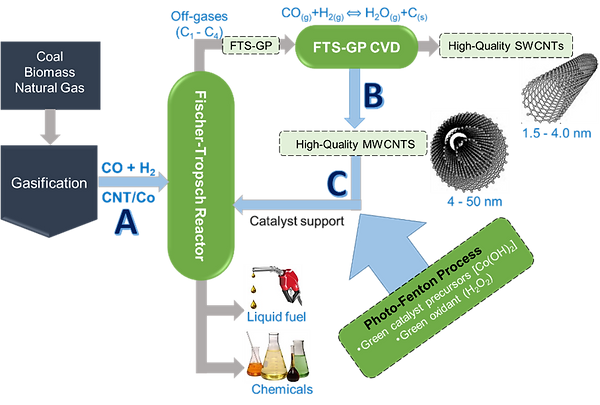
Figure 3. Schematic illustration of the closed-loop material concept: (A) using CNT-supported catalyst for FTS; (B) CVD growth of CNTs that use the waste gaseous mixture (FTS-GP) as a feedstock; and (C) using grown CNTs to produce efficient FT catalysts by modified photo-Fenton.
A modified photo-Fenton process is utilized for synthesis of CNT-supported catalysts. The process is facile, 'green,' and scalable, and provides a new pathway for controlled deposition of transition metal nanoparticles such as Fe or Co on CNTs. The process yields catalysts free of possible inhibitors typically present in conventional catalysts. For this reason, the modified photo-Fenton process does not require calcination, a post-synthesis treatment step that induces sintering or changes in the morphology. The process offers new opportunities for controlled synthesis of well-dispersed CNT-supported catalysts. These new CNT supports are expected to improve catalyst dispersion, mass transport of reactants and products, heat dissipation during the exothermic reaction, and catalyst stability.
Our Sponsors
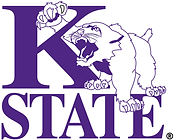

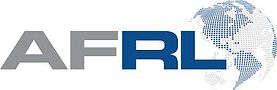